Nutrient Network (NutNet) Experiment
Objective:
The Nutrient Network (NutNet) is a globally distributed, bottom-up network of research sites and scientists interested in the impacts of nutrient enrichment on grassland ecosystems. Sites represent the regional flora (e.g. tallgrass prairie, desert grassland, alpine meadow, agronomic pasture, etc.) and are situated in a relatively homogeneous ~1000-m2 vegetation. By using a standardized experimental setup that is consistent across all study sites, NutNet addresses questions of whether plant diversity and productivity are co-limited by multiple nutrients and if so, whether these trends are predictable on a global scale. The NutNet site at SEV was started by the LTER in 2007.
Novelty:
Two of the most pervasive human impacts on ecosystems are the alteration of global nutrient budgets and changes in the abundance and identity of consumers. Fossil fuel combustion and agricultural fertilization have doubled and quintupled, respectively, global pools of nitrogen and phosphorus relative to pre-industrial levels. In spite of the global impacts of these human activities, there have been no globally coordinated experiments to quantify the general impacts on ecological systems. This has important implications for understanding how future atmospheric deposition of nutrients (N, S, Ca, K) might affect community and ecosystem-level responses.
Design:
At SEV, and globally, the fertilization treatments are applied at the scale of 5 m x 5 m plots (n=5 replicates) with 1m of buffer separation between every plot in a completely randomized design as follows: Three nutrient treatments (N, P and K plus micronutrients), each with two levels (control, added), are crossed in a factorial design, for a total of eight treatment combinations, to test multiple nutrient limitation on plant composition and ecosystem function. Nutrient addition rates and sources are: 10 gNm-2 year-1 as NH4NO3, 10 g P m-2 year-1 as triple-super phosphate [Ca(H2PO4)2], 10 g K m-2 year-1 as potassium sulphate [K2SO4] and 100 g m-2 micronutrient mix of Fe (15%), S (14%), Mg (15%), Mn (25%), Cu (1%), Zn (1%), B (02%) and Mo (005%). N, P and K are applied annually (prior to the monsoon season at SEV); micronutrients were applied once at the start of the experiment to avoid toxicity. Each plot contains one permanently located 1 m2 quadrat, marked at the corners with fiberglass stakes for measuring species composition and net primary production. The NutNet site was burned in the 2009 wildfire.
Responses:
Response variables include plant species composition; percentage ground cover of live perennial grasses, herbaceous dicots, shrubs, cactus, litter, and bare ground and aboveground net primary production. Soil parameters (organic matter content, pH, P, field available nitrogen (NO3-N and NH4-N), potentially mineralizable N) are measured occasionally. This experiment was initiated in May 2007 with one year of pre-treatment data. Nutrients are applied annually at the beginning of the monsoon season starting in 2008.
Supporting Documents:
Effects of Multiple Resource Additions on Community and Ecosystem Processes: NutNet NPP Quadrat Sampling at the Sevilleta National Wildlife Refuge, New Mexico. https://portal.edirepository.org/nis/mapbrowse?scope=knb-lter-sev&identifier=231
Data from SEV NutNet have been included in a large number of multi-site publications.
Risch, A.C., S. Zimmerman, R. Ochoa-Hueso, M. Schütz, B. Frey, J.L. Firn, P.A. Fay, F. Hagedorn, E.T. Borer, E.W. Seabloom, W.S. Harpole, J.M.H. Knops, R.L. McCulley, A.D.D. Broadbent, C.J. Stevens, P.B. Adler, J.V.S. Báez, L.A. Biederman, J.M. Blair, C.S. Brown, M.C. Caldeira, S.L. Collins, P. Daleo, A. di Virgilio, A. Ebling, E. Esch, A. Eskelinen, S. Guesewell, N. Hagenah, Y. Hautier, K.P. Kirkman, A.S. MacDougall, J.L. Moore, S.A. Power, S.M. Prober, C. Roscher, M. Sankaran, M.L. Speziale, P.M. Tognetti, R. Virtanen, L. Yahdjian and B. Moser. 2019. Soil net nitrogen mineralization across global grasslands. Nature Communications. https://doi.org/10.1038/s41467-019-12948-2.
Ochoa-Hueso, R., E.T. Borer, E.W. Seabloom, S.E. Hobbie, A.C. Risch, S.L. Collins, C.S. Brown, J. Alberti, H.A. Bahamonde, M.C. Caldeira, P. Daleo, C.R. Dickman, A. Ebeling, N. Eisenhauer, E. Esch, A. Eskelinen, S. Güsewell, B. Gutierrez-Larruga, K. Hofmockel, R. Laungani, E. Lind, A. López, R.L. McCulley, J. Moore, P. Peri, S.A. Power, J.N. Price, S.M. Prober, C. Roscher, J.M. Sarneel, M. Schütz, J. Siebert, R.J. Standish, S. Velasco Ayuso, R. Virtanen, G.M. Wardle, G. Wiehl, L. Yahdjian, T. Zamin. 2020. Microbial processing of plant remains is co-limited by multiple nutrients in global grasslands. Global Change Biology 26: 4572–4582. https://doi.org/10.1111/gcb.15146.
Hautier, Y., P. Zhang, M. Loreau, K.R. Wilcox, E.W. Seabloom, E.T. Borer, J.E.K. Byrnes, S.E. Koerner, K.J. Komatsu, J.S. Lefcheck, A. Hector, P.B. Adler, J. Alberti, C.A. Arnillas, J.D. Bakker, L.A. Brudvig, M.N. Bugalho, M. Cadotte, M.C. Caldeira, O. Carroll, M.J. Crawley, S.L. Collins, P. Daleo, L.E. Dee, N. Eisenhauer, A. Eskelinen, P.A. Fay, B. Gilbert, A. Hansar, F. Isbell, J.M.H. Knops, A.S. MacDougall, R.L. McCulley, J.L. Moore, J.W. Morgan, A.S. Mori, P.L. Peri, E.T. Pos, S.A. Power, J. Price, P.B. Reich, A.C. Risch, C. Roscher, M. Sankaran, M. Schütz, M.D. Smith, C. Stevens, P.M. Tognetti, R. Virtanen, G. Wardle, P.A. Wilfahrt and S. Wang. 2020. General destabilizing effects of eutrophication on grassland productivity at multiple spatial scales. Nature Communications 11. https://doi.org/10.1038/s41467-020-19252-4.
Baldarelli, L.M., H.L. Throop, S.L. Collins and D. Ward. 2021. Nutrient additions have direct and indirect effects on biocrust biomass in a long-term Chihuahuan Desert grassland experiment. Journal of Arid Environments. https://doi.org/10.1016/j.jaridenv.2020.104317
Seabloom, E.W., P.B. Adler, J. Alberti, L. Biederman, Y.M. Buckley, M.W. Cadotte, S.L. Collins, L. Dee, F.A. Fay, J. Firn, N. Hagenah, W.S. Harpole, Y. Hautier, A. Hector, S.E. Hobbie, F. Isbell, J.M.H. Knops, K.J. Komatsu, R. Laungani, A. MacDougall, R.L. McCulley, J.L. Moore, J.W. Morgan, T. Ohlert, S.M. Prober, A.C. Risch, M. Schuetz, C.J. Stevens and E.T. Borer. 2021. Increasing effects of chronic nutrient enrichment on plant diversity loss and ecosystem productivity over time. Ecology 102: e03218. https://doi.org/10.1002/ecy.3218
Sevilleta EDGE
Objective:
The Sevilleta Extreme Drought in Grasslands Experiment (SEV EDGE) examines the consequences of chronic drought and changes in precipitation seasonality on biodiversity and ecosystem services in both blue grama-dominated Plains grassland and black grama-dominated Chihuahuan Desert grassland ecosystems. The central question for EDGE is, How do community reordering and associated ecosystem functions recover from extreme drought? EDGE is a research platform that advances our understanding of patterns and mechanisms of ecosystem sensitivity to climate change, but it also benefits the broader scientific community by creating room for additional experiments and observations. With funding from the NSF Macrosystems Biology Program, EDGE initially was a geographically distributed field experiment established at six sites across NM, CO, WY, and KS. The two EDGE sites in the Sevilleta National Wildlife Refuge (SEV EDGE) are the hottest and driest in this distributed experiment. Ongoing operational support for SEV EDGE is now provided by the NSF LTREB program and LTER.
Regional climate predictions for the southwestern USA are dire – the probability of decadal droughts is nearly 100% by the end of the century (Cook et al. 2015). In addition, models predict less precipitation in July and August and more in September and October resulting in an extended period of aridity between spring rains and the start of the summer monsoon (Cook & Seager 2013). These predicted changes in precipitation amount and seasonality could have significant consequences for community reordering and ecosystem processes.
Novelty:
Although drought manipulations are common globally, few studies push systems beyond historical extremes, and fewer still impose the same treatment levels across different ecosystems and geographic locations. In addition, long-term experiments that address changes in precipitation seasonality are rare.
Design:
We manipulate rainfall amount and timing using a randomized block design with a total of thirty 3 x 4 m plots in each grassland ecosystem. Control plots (n=10) receive only ambient precipitation. Two rainfall treatments (n=10 each) are applied each year during the growing season: (1) rainout shelters (Yahdjian & Sala 2002) that remove 66% of growing season precipitation from April through mid-September, and (2) rainout shelters that capture and remove all precipitation in July and August and then reapply rain to the plots in September and October, using overhead sprinklers. This treatment extends the hyperarid period between spring and summer rains, effectively delaying the summer monsoon by two months.
Responses:
All EDGE replicates are instrumented with soil moisture, and soil and air temperature probes, and three replicates of each treatment at each site have soil CO2 probes. Soil CO2 concentration is measured at 15 min intervals with Vaisala CARBOCAP CO2 sensors (GMM222, Vaisala, Helsinki, Finland) placed at three depths (2, 8, and 16 cm). We calculate soil respiration using the flux-gradient method (Vargas et al. 2010). Aboveground net primary production (NPP) and plant species composition are now measured in two permanently located 1-m2 quadrats in spring and fall each year. Belowground NPP has been measured periodically in 5 replicates of each treatment with 5 cm diameter by 20 cm deep root in-growth bags during the monsoon season. Permanent root in-growth donuts were installed in 2020 to measure belowground NPP from 0-30 cm depth in the soil.The drought treatment ended in 2020, and those plots are now recovering from drought. The delay treatment is ongoing.
Supporting Documents:
Extreme Drought in Grassland Ecosystems (EDGE) Seasonal Biomass and Seasonal and Annual NPP Data at the Sevilleta National Wildlife Refuge, New Mexico. https://portal.edirepository.org/nis/mapbrowse?scope=knb-lter-sev&identifier=298
Cook, B. I., T. R. Ault, and J. E. Smerdon. 2015. Unprecedented 21st century drought risk in the American Southwest and Central Plains. Science Advances 1:e1400082–e1400082. https://doi.org/10.1126/sciadv.1400082
Cook, B. I., and R. Seager. 2013. The response of the North American Monsoon to increased greenhouse gas forcing. Journal of Geophysical Research: Atmospheres 118. https://doi.org/10.1002/jgrd.50111
Fernandes, V. M. C., N. M. Machado de Lima, D. Roush, J. A. Rudgers, S. L. Collins, and F. Garcia-Pichel. 2018. Exposure to predicted precipitation patterns decreases population size and alters community structure of cyanobacteria in biological soil crusts from the Chihuahuan Desert. Environmental Microbiology 20:259–269. https://doi.org/10.1126/sciadv.1400082
Griffin-Nolan, R.J., D.M. Blumenthal, S.L. Collins, T.E. Farcas, A.M. Hoffman, K.E. Mueller, T.W. Ocheltree, M.D. Smith, K.D. Whitney and A.K. Knapp. 2019. Shifts in plant functional composition following long-term drought in grasslands. Journal of Ecology 107: 2133–2148. https://doi.org/10.1111/1365-2745.13252
Lagueux, D., A. Jumpponen, A. Porras-Alfaro, J. Herrera, Y.A. Chung, L.A. Baur, M.D. Smith, A.K. Knapp, S.L. Collins and J.A. Rudgers. 2021. Experimental drought re-ordered assemblages of root-associated fungi across North American grasslands. Journal of Ecology 109: 776-792. https://doi.org/10.1111/1365-2745.13505
Ochoa-Hueso, R., S.L. Collins, M. Delgado-Baquerizo, K. Hamonts, W.T. Pockman, R.L. Sinsabaugh, M.D. Smith, A.K. Knapp and S.A. Power. 2018. Drought consistently alters the composition of soil fungal and bacterial communities in grasslands from two continents. Global Change Biology 24: 2818–2827. https://doi.org/10.1111/gcb.14113
Shi, Z., M. L. Thomey, W. Mowll, M. Litvak, N. A. Brunsell, S. L. Collins, W. T. Pockman, M. D. Smith, A. K. Knapp, and Y. Luo. 2014. Differential effects of extreme drought on production and respiration: synthesis and modeling analysis. Biogeosciences 11:621–633. https://doi.org/10.5194/bg-11-621-2014
Yahdjian, L., Sala, O.E. A rainout shelter design for intercepting different amounts of rainfall. Oecologia 133, 95–101 (2002). https://doi.org/10.1007/s00442-002-1024-3
Soil Disturbance to Biocrusts
Objective:
This project tests how soil disturbances that reduce biocrusts affect the composition of dryland communities. Over the long term, it will provide a window on how disturbances to biocrusts may influence the sensitivities of plants and ecosystem processes to climate change and increasing climate variability. The work addresses the LTER core research area to understand how disturbances affect ecological dynamics. Information will help to interpret the potential community and ecosystem-level impacts of on-going climate experiments that reduce biocrusts because here, we directly manipulate biocrust abundance through manual disturbances to the soil surface.
Novelty:
The loss of biological soil crusts (biocrusts) is occurring in dryland ecosystems worldwide. Causes include land use intensification, grazing, human disturbance of soils (trails, ATVs), and climate change. Consequences of the loss of biocrusts include altered soil surface hydrology, changes to soil C and N dynamics, and increased soil erosion and dust production. Past experiments worldwide have largely focused on the small scale of biocrusts with experiments that isolate drivers of their abundance, or the large scale of landscapes to quantify the roles of biocrusts in soil erosion and dust production. Much less is known about the changes in community composition that may accompany losses of biocrusts. Our experiments have potential to expand current knowledge on the biological importance of biocrusts for dryland communities and ecosystem processes.
Design:
This project is located in three ecotones at the Sevilleta National Wildlife Refuge: a mixed creosote shrubland-black grama grassland site, a mixed blue grama-black grama grassland, and a piñon-juniper woodland to juniper savanna transition zone. At each site, we have 20 plots (6 m X 6 m) with similar initial plant and biocrust cover and plant species composition (10 disturbed plots and 10 controls per site). To determine potential impacts of the loss of biocrusts, we impose soil disturbance that reduce biocrust abundance twice yearly, after data collection in May and October. Our treatment disturbs all non-vegetated soil surfaces to a depth of ~ 5cm. Control plots are not disturbed. Disturbance involves two personnel wearing thickly treaded boots, each stepping on and scuffing up all non-vegetated soil surfaces in the plot. Using this method, each non-vegetated patch in the plot receives two “stomps” on each treatment date. The disturbance treatment was assigned to plots in a completely randomized design, and beginning June 2013 (May 2018 for PJ), disturbance was imposed twice each year (May, Oct) to capture the beginning and end of the summer monsoon season. Shrubland and grassland sites began Sept 2013, piñon-juniper was added in May 2018 as part of the new LTER program.
Responses:
We continuously monitor plant species composition and biomass in two permanent 1 m X 1 m quadrats per plot. Plant data collection transferred to the SEV LTER Field Crew in 2018. Twice yearly, we archive roots and rhizosphere soils from blue grama and black grama, collect soil cores for gravimetric soil moisture, and 0-2cm deep biocrust samples for pigment analysis of chlorophyll and scytonemin. Less frequently measured response variables include soil texture, infiltration, nutrient analyses, soil organic matter, and C:N ratios of leaves of blue grama and black grama.
Supporting Documents:
Chung, Y. A., B. Thornton, E. Dettweiler-Robinson, and J. A. Rudgers. 2019. Soil surface disturbance alters cyanobacterial biocrusts and soil properties in dry grassland and shrubland ecosystems. Plant and Soil 441:147–159. https://doi.org/10.1007/s11104-019-04102-0
Warming- El Nino- Nitrogen deposition Experiment
Objective:
The question addressed by WENNDEx is, How do multiple global change drivers interact to affect the rate at which species reordering occurs, and what changes in ecosystem functioning are associated with this reordering? Answers to this question can elucidate how ecological presses interact to shift species dominance and affect sensitivity of key components of the C cycle to global change drivers and natural, interannual variation in climate. WENNDEx was built in 2006 with funding from NSF-DEB Ecology Program; operational support is now provided by the NSF LTREB program, in addition to LTER.
Multiple global change drivers will impact community and ecosystem structure and function during the next century. Many of these environmental drivers are subtle and persistent presses, such as changing precipitation regimes, increasing temperature, or atmospheric N deposition, with impacts that accumulate over time. These chronic environmental drivers directly and indirectly alter resource availability and interspecific interactions. Predicting how multiple global change drivers will interact is challenging because the effects of co-occurring environmental changes may or may not be additive, and these effects can change or cancel out over decadal time periods. The purpose of WENNDEx is to better understand the potential interactions among environmental changes for grassland community composition, ecosystem processes, and the recruitment of creosotebush seeds and seedlings to estimate the probability of a future state transition to a shrubland ecosystem.
Novelty:
Major changes in terrestrial ecosystems include warming, nitrogen deposition, and altered precipitation regimes. Most field experiments focus on single factors, ignoring the potential for interactions among environmental change factors. Tests of interactions among co-occurring environmental changes are critical to predicting our future and improving management strategies. Over the long-term, we can also evaluate the potential for treatments to interact with increases in both aridity and interannual precipitation variability because this experiment occurs in a highly variable, and drying background climate.
Design:
This multi-factorial experiment has three fully crossed factors: nighttime warming, winter water addition, and nitrogen addition in a completely randomized design for a total of eight treatment combinations. There are five replicates for each of the eight treatment combinations, for a total of 40 plots. Plots are 3 m x 3.5 m. All replicates contain both blue grama and black grama grass. Nighttime warming is imposed on the full plot using lightweight aluminum fabric blankets that are drawn across each warmed plot at night to trap outgoing longwave radiation. Dataloggers controlling shelter movements retract the blankets when wind speeds exceed a threshold (to prevent damage) and when rain or snow occurs. Based on long-term climate records, El Niño rains increase average winter precipitation in our area by 50%, and more intense El Niño events are predicted by climate models. During El Niño winters only, we supplement ambient winter precipitation using an irrigation system and reverse osmosis (RO) water. Rain is added in six experimental events during treatment years (January-March) to mimic actual El Niño winter-storm event size distribution (four 5 mm events, one 10 mm event, and one 20 mm event each winter) and amount (50 mm). Using a backpack sprayer we add 2 g N m-2 y-1 as NH4NO3 prior to the monsoon season because NH4-N (57%) and NO3-N (43%) contribute approximately equally to N deposition at SNWR. Control plots receive the same amount of RO water.
Burn: On August 4, 2009, a lightning-initiated fire began on the Sevilleta National Wildlife Refuge. By August 5, 2009, the fire had reached the WENNDEx site, which was burned extensively though not entirely. Approximately 50% of plots burned on August 5, and those plots which did not burn were burned within three weeks by US Fish and Wildlife Service. Thus, the condition of all plots at the WENNDEx site was comparable by early September 2009.
Responses:
Aboveground net primary production (NPP) and plant species composition are measured allometrically in two 1-m2 permanent subplots in each treatment plot, twice yearly. We measure temperature in all plots at two soil depths (5 cm, 10 cm) and 20 cm aboveground with Campbell Scientific CS107 temperature probes. Soil moisture is measured in each plot using Campbell Scientific CS-616 probes buried at a 45o angle to obtain an integrated measure of moisture in the top 20 cm. Moisture and temperature probes take a reading every 15 minutes. Soil N availability is measured during the monsoon (July – Sept) during some years using Plant Root Simulator probes (WesternAg Industries, Saskatoon, Canada) placed in either blue grama or black grama patches in each plot. Following the fire, we began measurements of soil respiration in a subset of treatments (control, warmed, winter rain, and warmed plus winter rain) measured at 15 min intervals with Vaisala CARBOCAP CO2 sensors (GMM222, Vaisala, Helsinki, Finland) placed at three depths (2, 8, and 16 cm), and with sensors under the canopy of either blue grama or black grama.
We focus on three dominant plant species, all of which are near their range margins, and thus may be particularly susceptible to interacting environmental changes. We hypothesize that warmer summer temperatures and increased evaporation will favor growth of black grama (Bouteloua eriopoda), a desert grass, but that increased winter precipitation and/or available nitrogen will favor the growth of blue grama (Bouteloua gracilis), a shortgrass prairie species. Furthermore, growth and survival of the native shrub creosote (Larrea tridentata, added as seeds and seedlings) may be promoted by heightened winter precipitation, N addition, and/or warmer nighttime temperatures. Treatment effects on limiting resources (soil moisture, nitrogen mineralization), species abundance, and above- and belowground net primary production (NPP) are all being measured to determine the interactive effects of key global change drivers on arid grassland plant community dynamics.
Supporting Documents:
Collins, S. and W. Pockman. 2020. Warming-El Nino-Nitrogen Deposition Experiment (WENNDEx): Soil Nitrogen Data from the Sevilleta National Wildlife Refuge, New Mexico (2006 – 2020). https://portal.edirepository.org/nis/mapbrowse?scope=knb-lter-sev&identifier=307
Warming-El Nino-Nitrogen Deposition Experiment (WENNDEx): Net Primary Production Quadrat Data at the Sevilleta National Wildlife Refuge, New Mexico. https://portal.edirepository.org/nis/mapbrowse?scope=knb-lter-sev&identifier=176
Warming-El Nino-Nitrogen Deposition Experiment (WENNDEx): Soil Temperature, Moisture, and Carbon Dioxide Data from the Sevilleta National Wildlife Refuge, New Mexico. https://portal.edirepository.org/nis/mapbrowse?scope=knb-lter-sev&identifier=305
Collins, S.L., J.E. Fargione, C.L. Crenshaw, E. Nonaka, J.R. Elliott, Y. Xia and W.T. Pockman. 2010. Rapid plant community responses during the summer monsoon to nighttime warming in a northern Chihuahuan Desert grassland. Journal of Arid Environments 74: 611-617. https://doi.org/10.1016/j.jaridenv.2009.10.005
Collins, S.L., L.M. Ladwig, M.D. Petrie, S.K. Jones, J.M. Mulhouse, J Thibault and W.T. Pockman. 2017. Press-pulse interactions: Effects of warming, N-deposition, altered winter precipitation and fire on desert grassland community structure and dynamics. Global Change Biology 23: 1095-1108. https://doi.org/10.1111/gcb.13493
Stochastic Dynamic Program (SDP)
Objective:
To develop a predictive understanding of both foraging and population dynamics within the Sevilleta small mammal community, the physiological and environmental conditions driving alternative foraging behaviors among both caching and non-caching rodent species are incorporated into a mechanistic state-dependent model. Our stochastic dynamic program (SDP) determines how species-specific body condition and caching behaviors, as well as environmental uncertainty (e.g., plant productivity), impacts foraging. The goals of our SDP are threefold, and involve determining: 1) which resource type (C3 vegetation, C3 seeds, C4 vegetation, C4 seeds, or insects) maximizes an individual’s fitness with respect to its age and its current endogenous and exogenous (cache) energetic state, 2) whether a given resource should be consumed or cached, and 3) the population-level consequences of the predicted strategies.
Novelty:
When combined with the novel data collected during our monthly small mammal trapping program, the stochastic dynamic program will generate predictions of foraging behavior based on the resource landscape as well as endogenous and exogenous (cached) energy stores. This model will be used to project the dynamics of populations based on fitness-maximizing foraging strategies that directly affect individual reproduction and survival. This process will enable us to forecast extinction risk under environmental conditions anticipated by future climate scenarios.
Design:
In our framework, an individual must balance maintaining its body condition while establishing a buffer of endogenous and/or exogenous reserves to survive a stochastically varying environment. Foraging decisions maximize the individual’s future fitness integrated over the course of its lifetime, thus predicting behaviors representative of the evolutionary endpoint of natural selection. As such, the SDP framework will be used to assess the impact of fitness-maximizing foraging behaviors on population dynamics, ultimately linking the effects of different resource distributions to extinction risk.
Responses:
We will inform the SDP with empirical data describing the relationship between body condition and reproductive output, such that the fitness values associated with decision arrays will permit simulations of individual birth/mortality through time, allowing us to calculate population size, survival, extinction risk, and the presence or absence of cycles. To what extent these model outputs conform to empirical observations will be assessed both qualitatively and quantitatively.
Supporting Documents:
Yeakel, J.D., Bhat, U., Newsome, S.D. (2020) Caching in or falling back at the Sevilleta: the effects of body size and seasonal uncertainty on desert rodent foraging. American Naturalist.
Terrestrial ECOsystem Model (TECO)
Objective:
We are developing a new dryland version of the ecosystem model TECO (Weng & Luo 2008). This model assimilates SEV LTER data to constrains predictions on ecosystem responses to climate trends and variance. We use the TECO model to predict ecosystem dynamics into the future and explore parameter space beyond what we can manipulate. These efforts allow us to explore the responses of biogeochemical processes and biophysical feedbacks and predict ecosystem responses to climate variability following disturbances.
We intend to build a new front-end to TECO to link traits to ecosystem processes, increasing information on why are some ecosystems are more sensitive to climate mean and variance than others. By varying trait distributions in TECO simulations, we can evaluate how trait change alters ecosystem sensitivity to climate over long time periods. We have the distinct advantage of 10+ years of ongoing tower flux data from each SEV ecosystem type, which aid in both parameterization and validation of TECO.
Novelty:
Prior work has focused on predicting ecosystem processes responses to changing mean climate. Our SEV LTER program adds novelty by considering the mean × variance interaction, which is particularly relevant for drylands. Moreover, TECO will be first constrained by observations via data assimilation before used to predict ecosystem responses to changing mean and variance of precipitation.
Design:
TECO is a typical terrestrial ecosystem model that simulates biophysical and biogeochemical processes in ecosystems. TECO has five major components: (1) canopy photosynthesis, (2) soil water dynamics, (3) plant growth, (4) litter and soil C decomposition and transformation, and (5) N dynamics. Canopy photosynthesis is derived from a two-leaf (sunlit and shaded) model that simulates canopy conductance, photosynthesis, and partitioning of available energy. In the soil water dynamic submodel, soil is divided into 10 layers. Soil water content of these layers is determined by the mass balance between water influx and efflux. The plant growth submodel simulates carbon allocation and phenology. Allocation of the carbon among different plant components depends on their growth rates. Phenology is related to leaf onset, which is triggered by growing degree days, and leaf senescence, which is determined by temperature and soil moisture. The transfer submodel estimates carbon transferring from plants to litter and three soil layers. The nitrogen submodel is fully coupled with carbon processes with one additional mineral N pool. N is absorbed by plants from mineral soil and partitioned among leaf, woody tissues, and fine roots. N in plant detritus is transferred among different ecosystem pools (i.e. litter, fast, slow and passive SOM). The model is driven by climate data (including air and soil temperature, vapor-pressure deficit, relative humidity, incident photosynthetically active radiation, and precipitation) at hourly steps.
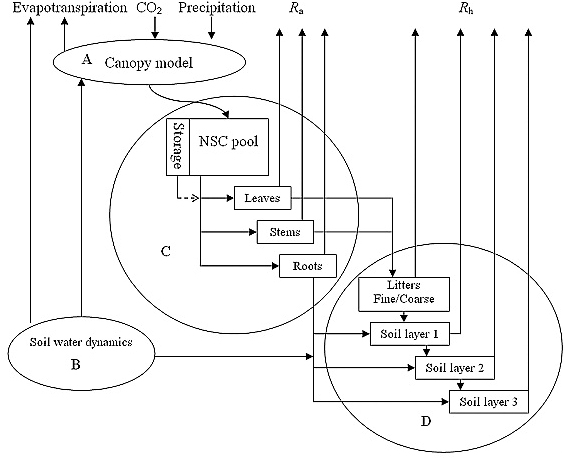
Soil hydrological properties regulate grassland ecosystem responses to multifactor global change: A modeling analysis.
Responses:
TECO has been used successfully to quantify the main and interactive effects of climate warming, altered precipitation, and elevated [CO2] on net primary production (NPP), heterotrophic respiration (Rh), net ecosystem production (NEP), transpiration, and runoff in a variety of ecosystems (e.g., Luo et al. 2008, Xu et al. 2015a). In addition, we have used TECO in model inter-comparison projects with a dozen other ecosystem models at 22 AmeriFlux sites and several CO2 experiments. Various datasets, including data streams for meteorological data, water table, phenology, and respiration, plus discrete measurements of C flux, above- and belowground biomass, and soil carbon content, have been assimilated through EcoPAD to constrain the TECO model. A distinct advantage of TECO is that it can be used effectively in a data assimilation (DA) framework. Luo and his group have used TECO with data assimilation in many other ecosystems and experiments.
In this project, TECO will be wrapped into EcoPAD to assimilate observations from the mean x variance experiment and forecast ecosystem responses to experimental treatments in a near-real-time fashion so as to advance our dryland research via timely interactions between experimenters and modelers.
WAVE: A Modeling Framework for Habitat Ecotones
Objective:
A broad objective of the Sevilleta LTER is to understand the trajectories of ecosystem transition zones under current and future climate scenarios. The WAVE model provides a quantitative framework for combining demographic responses to climate, interspecific interactions, and seed movement to determine the direction and pace of change at ecotones between foundation plant species.
Novelty:
State transitions are often studied at the ecosystem scale without explicit consideration of the lower-level process that give rise to ecosystem-level phenomena. The WAVE model captures the building blocks of state transitions — births, deaths, and movements of foundation species — and can integrate with TECO to link demographic processes with ecosystem-level process outcomes
Design:
The WAVE model is based on integrodifference equations, which have a long history in spatial ecology. These models predict the growth of populations in space and time based on the combined effects of demography (which determines change in local density) and dispersal (which determines the spatial redistribution of propagules). The WAVE model couples the dynamics of two foundation-species populations, generating a collision ecotone where they intersect. The trajectory of the ecotone — whether it persists stably or moves directionally, with one species invading the other — depends on the combined effects of local density-dependent demography (intra- and inter-specific interactions) and seed dispersal. The WAVE model is designed to accommodate data inputs from results of our Mean – Variance Experiment, allowing us to predict ecotone dynamics under climate scenarios with altered mean and variance of soil moisture.
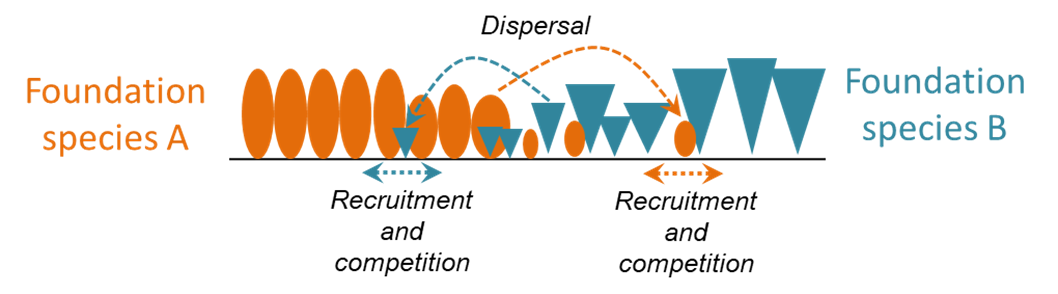
The WAVE model provides a quantitative framework for combining demographic responses to climate, interspecific interactions, and seed movement to determine the direction and pace of change at ecotones between foundation plant species.
Responses:
The WAVE model predicts the velocities of expansion/contraction for two foundation plant species at their ecosystem transition zone. From these velocities, we can derive estimates for ecotone stability or the direction and pace of transition.
Carbon, Water, and Energy Fluxes: New Mexico Elevation Gradient
Objective:
Quantifying carbon, energy and water balance in ecosystems and understanding the processes regulating them is crucial to effective policy decisions and public understanding of climate change science.
Novelty:
Dryland ecosystems occupy ~45% of global land surface and contain almost twice the carbon stored in temperate forest ecosystems. Despite the large area and carbon stores in these ecosystems, there are large uncertainties on their carbon budgets, due to a lack of research. To reduce these uncertainties, the New Mexico Elevation Gradient of eddy covariance flux towers, directed by Dr. Marcy Litvak and supported by Ameriflux uses these long-term records to, and fill gaps in understanding of the mechanisms regulating carbon, energy and water fluxes in dryland ecosystems of the Southwestern USA .
Design:
Eddy covariance flux towers are located in nine sites, distributed across a 1500 m gradient in elevation, climate and land cover that we refer to as the New Mexico elevation gradient (NMEG). The towers span six ecosystems: C4 dominant grassland, creosotebush shrubland, juniper savanna, piñon‐juniper woodland, ponderosa pine forest and subalpine mixed conifer forest, which collectively represent ~55% of the land cover of the four corner states in the southwestern US. The towers also allow us to quantify both the short and long-term impacts of dominant disturbances occurring in the southwestern USA on ecosystem processes: drought, fire, bark beetle outbreak, spruce budworm outbreak, and extreme freeze events.
Responses:
Continuous, multi‐year observations (since 2007) across this network have allowed us to identify the primary mechanisms regulating carbon, water and energy exchange across a wide range of semi‐arid ecosystems. We also use these datasets to examine how long-term patterns in carbon sequestration and water use vary with changes in climate, vegetation, soil characteristics, and disturbance regime.
Supporting Documents:
Learn More and Access Data
Sevilleta Sites
Sites East of Sevilleta
Chemical Defense Investment Under Drought Stress
Objective:
Building on the initial piñon-juniper drought experiment (see Historical Projects, Piñon-Juniper Drought Experiment 1.0), this project seeks to quantify links between plant physiological and defense strategies in mature trees and elucidate the underlying mechanisms of these responses over a range of drought conditions. This work is primarily supported by a separate NSF DEB award to Pockman.
Novelty:
This work seeks a mechanistic understanding of how tree drought stress affects the interactions among hydraulic function, carbohydrate availability, and chemical defense, including constitutive and induced defense components. The piñon-juniper system has been widely studied as a model of drought-induced plant mortality. This project addresses the paucity of information about the role of plant defensive responses during drought, particularly the existence of thresholds of drought causing diminished defense against bark beetles, their symbiotic fungi and other pathogens.
Design:
This experiment builds on the Piñon-Juniper Drought Experiment 1.0 by using the same basic design of large scale plots that intercept ambient precipitation and prevent it from reaching the plots in order to reduce plant-available water. Whereas the initial experiment imposed a replicated drought treatment that reduced ambient precipitation by ~45%, this experiment used the same infrastructure to impose different levels of drought along a gradient (45%, 70%, and 90%, in addition to a ‘legacy’ 45% plot established in 2010 during the first experiment).
Responses:
We measure plant water potential, micrometeorology, non-structural carbohydrates (NSC), enzyme activities, carbon isotope composition of NSCs, and emissions of volatile organic compounds to assess plant defensive responses across treatments.
Desert small mammal populations long-term monitoring program
Objective:
Desert consumers are integral to the ecosystems of the Sevilleta. We examine how rodent diversity and abundance change over time, tracking climate and plant-based resources since 1989.
Novelty:
Our long-term trapping program has continuously monitored small mammals at the Sevilleta National Wildlife Refuge, where distinctive ecosystems collide. These data provide some of the world’s longest continuous records on dryland rodent communities. Current monitoring includes core sites in Chihuahuan Desert grassland, Plains grassland, and Desert shrubland dominated by creosotebush, with data extending back to 1989. Prior, shorter-term monitoring included sites in piñon-juniper woodlands, other grassland and shrubland locations, and grass-shrub transition zones.
Design:
Small mammal trapping and data collection occur twice yearly, during spring and fall to capture pre-monsoon and post-monsoon changes in the population dynamics of dryland rodents. We monitor 31 small mammal species, with data spanning 8 sites (3 sites are current).
Responses:
Each rodent captured is identified to species, sexed, assessed for life stage, weighed, and then returned to the site of capture. Our web sampling design consists of 148 traps placed in a concentric circle (200m diameter) composed of 12 traps each on 12 spokes with four traps located at the center of the web facing all four cardinal directions. Sherman traps are baited nightly for three consecutive nights. Each core site has 5 replicate trapping webs.